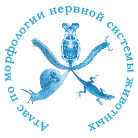

Brief overview of the architecture and
|
||
AuthorEditor |
The early evolution of the chordates (Chordata) took place in the Precambrian, when the primitive tunicates (subphylum Tunicata) had undergone neoteny and lost their sessile stage. The primitive tubular nervous system has evolved early on in the ancestral lineage of the chordates and emerged in evolution all at once as an integrated whole. In ascidians, the most typical members of the subphylum Tunicata, the neural tube develops in the long tail region of the larva above the notochord and the gut. The anterior end of the neural tube is enlarged to form the so-called cerebral vesicle, which is connected with the primitive sense organs: multicellular eyespot and unicellular organ of gravity (statocyst). Later in their development, the ascidians undergo the regressive metamorphosis, which involves the transition of adult animals to the “sessile” mode of life on the sea bottom. During the metamorphosis, the major part of the neural tube becomes reduced and the adult animal retains only the suprapharyngeal (cerebral) ganglion that develops from the dorsal roof of the larval cerebral vesicle. |
Contents
|
The origin of the tubular nervous system |
In all chordates, the primordial nervous system is located on the dorsal side of the embryo where it forms the neural plate above the developing notochord. The origin of the tubular nervous system in acraniates (subphylum Acrania) was associated with the development of the axial musculature, i.e. the “myo-notochord complex”, which is innervated from intraepithelial nerve nets. These primary intraepithelial cells become separated from the surface epithelium and submerge into the body wall of the trunk to form the primary neural plate. Subsequently, the lateral edges of the neural plate grow thicker and bulge over the surface of the plate as a pair of neural (or medullar) folds, while the middle portion of the neural plate curves inward to form the neural groove along the dorsal side of the embryo. The neural folds then grow upward and towards the midline and fuse with each other transforming the groove into the neural tube. The cells of the surface ectoderm lying on either side of the neural groove begin to divide and grow towards the midline and the ectoderm closes up over the neural tube. The ectodermal origin of the neural tube is also supported by the fact that pores at the rostral and caudal poles of the vertebrate embryos through which the cavity of the neural tube communicates to the exterior (neuropores) remain open for a long period of time. The tubular nature of the nervous system proved to be so efficient that it remained essentially unchanged throughout the evolutionary history of chordates. |
|
The origin of the brain |
In 1868 F.V. Ovsyannikov has demonstrated that the differentiation of the neural tube into the cephalic and trunk regions has already begun in evolution as early as in the cephalochordates represented today by lancets. This evolutionary event was associated with the concentration of the primary receptor structures and their centers at the anterior end of the body in ancient cephalochordates. Later in the history of vertebrates, the acraniates have been replaced by ancient craniates (Craniata), which already had well-developed sense organs together with the differentiated brain and spinal cord. Vertebrates are subdivided into two large branches: jawless vertebrates (Agnatha) represented today by a single class Cyclostomata, and jawed vertebrates (Gnathostomata), which include all other vertebrates. The ancestral group for both lineages were telodonts (subclass Thelodonti of the class Pteraspidomorphi), small armorless forms, which inhabited the coastal sea regions and lagoons. Primitive agnathans lived on the bottom and were migratory inhabitants of estuaries and lagoons. The brain of the ancient agnathans was elongated along one plane, the olfactory lobes were not separated from the forebrain, the cerebellum and diencephalon were asymmetrical and already relatively well-developed. The hindbrain was associated with the octavo-lateralis sensory system, the midbrain with the optic system and the forebrain with the olfactory system. Thus, at this early stage of vertebrate evolution the brain has already been differentiated into several regions, whose origin and evolution appear to be closely associated with the emergence of systems of remote sensing (most notably the vision and the olfactory system), which were essential for spatial orientation. |
|
Cyclostomes |
Cyclostomes (Cyclostomata) have originated from Anaspida, nektonic armorless agnathans, some of which were protected only by keratinous scales and were adapted to use the bodies of dead armored agnathans as temporary shelters and sources of food. One of the descendant groups of these animals, the lampreys (subclass Petromyzones), have adopted the ectoparasitic and semipredatory lifestyle in fresh and brackish waters, while the other group, the hagfishes (subclass Myxini), has evolved a special form of predation at sea. These two lineages have emerged before the Carboniferous. This long evolutionary path from Anapsida to the typical lampreys and especially to the hagfishes may have resulted in a certain secondary simplification in the structure and function of their nervous system. The cyclostomatans, however, were the first class of vertebrates that had all five major regions of the vertebrate brain (Fig. 1). |
|
Brain regions in the vertebrate animals |
The rostral region of the neural tube develops into the telencephalon, which is followed posteriorly by the diencephalon and then by the midbrain (mesencephalon). The primary hindbrain differentiates into the secondary hindbrain (metencephalon) and myelencephalon (medulla oblongata), which transitions into the spinal cord (medulla spinalis) (Fig. 2).
Fig. 2. Schematic representation of the brain in lower and higher vertebrates (after Andreeva, Obukhov, 1999): vascular beds of the telencephalon (1), diencephalon (2 and 3), and the hindbrain (4); 5 – parapineal organ, 6 – pineal organ; colliculi superiores (7) and colliculi inferiores (8) of the midbrain; VIII and VIV – ventricles of the diencephalon and the hindbrain; olfactory (I) and optic (II) cranial nerves; BO – olfactory bulb, Tel – telencephalon, Crx – cortex of telencephalic hemispheres, cc – corpus collosum, Str – striatum (basal ganglions), Hy – hypothalamus, hyp – hypophysis, Th – thalamus, E – pineal gland, Mes – mesencephalon, Tg – tegmentum, Pn – pons, Mo – myelencephalon, TO – tectum opticum, Msp – spinal cord, Pal – cortical formations of the telencephalon in lower vertebrates (pallium).
The spinal cord (Medulla spinalis) is formed from the caudal regions of the neural tube. It is a part of the central nervous system (CNS), whose structure retains the traits of the embryonic stages of the vertebrate brain, the tube-like shape and the segmental structure. Myelencephalon (Medulla oblongata) is the caudal-most region of the brain that gradually transitions into the spinal cord. The conventional boundary line between the brain and the spinal cord is at the roots of the first pair of spinal nerves. The most significant similarity with the spinal cord is observed in the caudal regions of the myelencephalon; this similarity is found in the arrangement of grey and white matter and in the central position of the brain canal. The canal widens in the rostral direction to form a diamond-shaped or triangular cavity, the 4th ventricle (ventriculus quartus). The roof of the ventricle is thin and consists only of the ependymal epithelium overlain in higher vertebrates by a highly vascularized pia matter. In lower vertebrates, grey matter is composed of strands, or columns, of nerve cells that lie along the myelencephalon, while in the higher vertebrates these strands tend to split into individual nuclei. The hindbrain (metencephalon) is represented in all vertebrates by the cerebellum, whose shape, size and constituent parts vary significantly in different vertebrate groups and generally correlate with the degree of sophistication of their motoric reactions. In mammals, a large system of nerve tracts and nuclei that forms an independent region called pons (bridge) has evolved in the ventral and lateral walls of the hindbrain, which are poorly developed in lower vertebrates. The cavity of the hindbrain is formed by the rostral regions of the 4th ventricle. Midbrain (mesencephalon). The midbrain consists of a derivative of the alar plate (the roof of the midbrain, tectum mesencephali), and a derivative of the basal plate (the midbrain tegmentum, tegmentum mеsencephali). In higher vertebrates, with the emergence of new brain regions and their connections, the structures of the corpora quadrigemina ("quadruplet bodies"): colliculi superiores et inferiores, have evolved at the place of the optic lobes. Another structure that have formed in higher vertebrates are the cerebral peduncles (pedunculi cerebri), which constitute a system of nerve tracts connecting the higher regions of the CNS to its lower regions. The cavity of the mesencephalon is formed by the remains of the cavity of the middle cerebral vesicle, the cerebral aqueduct (aqueductus cerebri). The diencephalon comprises the dorsally located epithalamus, the thalamus that occupies the central portion of the diencephalon and the ventral hypothalamus. In higher vertebrates, the diencephalon also includes the subthalamus, which houses large nerve centers: lateral and medial geniculate bodies (corpus geniculatum laterale et medialis). It should be noted that the thalamus has undergone a number of structural transformations towards higher vertebrates caused primarily by the development of associations between this brain region and the progressively evolving telencephalon. The telencephalon is the most complex region of the brain. In different vertebrate groups, the telencephalon has been evolving along two different paths (see below). The telencephalic hemispheres reach their highest level of sophistication in higher mammals: primates and humans. The telencephalon consists of the dorsal pallial (Pallium) and ventral subpallial (Subpallium) regions. The pallium comprises cortical formations subdivided into the ancient (Paleocortex), old (Archicortex) and new (Neocortex) cortices. Like the cortex, the subcortical formations have a long evolutionary history and consist of phylogenetically different regions: paleostriatum, archistriatum and neostriatum. |
|
Theory of critical periods |
In the 1950–1970s, A.I. Karamyan, eminent Soviet evolutionary neurophysiologist and a corresponding member of the Soviet Academy of Sciences, who was a disciple of L.A. Orbeli and a proponent of his ideas, proposed a theory of crucial (critical) periods in evolution of the vertebrate CNS. The premise of this theory was that the evolution of the brain in vertebrates has progressed from diffuse, non-specialized forms to specialized forms of structural and functional organization. During certain periods of vertebrate evolution (“critical periods”, in Karamyan’s terminology), the degree of CNS specialization shows a strict correlation with the level of conditional reflex activity. This process proceeds through the “buildup” of phylogenetically young, progressive CNS regions over “ancient” brain regions that tend to lose some of their functions and associations (Fig. 3). The study of the structural and functional organization of the nervous system of the lancelet and cyclostomes places these vertebrate groups within the first critical stage of the CNS evolution (Fig. 3a, b). As noted above, the lancelet shows almost no signs of cephalization. Its central nervous system has a diffuse organisation and the brain as a CNS region has yet to be fully formed. This level of integration is called the spinal integration. The cyclostomes (lampreys), unlike agnathans, have already developed all major brain regions, but they still retain some elements of the diffuse organization of the brain. Since the myelencephalon and the midbrain are the most differentiated brain regions in cyclostomes, this stage is called the bulbo-mesencephalic integration. The other critical stages in the evolution of the CNS are described below, in the sections devoted to the respective groups of vertebrates. |
|
First jawed vertebrates (Protognathostomata) |
The transformation of the first gill arches into jaws, which served for active prey capture, and the development of paired fins gave the first jawed vertebrates a significant evolutionary advantage over the agnathans. The ability for active feeding has dramatically enhanced their vital functions, allowed them to conquer the aquatic environments and gave rise to one of the largest and the most diverse vertebrate superclasses, the Fishes (Pisces). Placoderms (Placodermi) is the most ancient and now entirely extinct class of fishes. Cartilaginous fishes (class Chondrichthyes) first appear in the fossil record in the early Devonian. This group retained more ancestral traits in their morphology than did the placoderms. Elasmobranchs (subclass Elasmobranchii) are the largest group of cartilaginous fishes that were quite abundant in the Paleozoic in the brackish waters of seas and lagoons, and during the Cenozoic have spread into the seas and oceans. Elasmobranchs are subdivided into sharks and rays, which represent different stages of fish evolution. Elasmobranchs with flattened (“skate-shaped”) bodies have arisen more than once from the shark-like ancestors. The evolutionary conserved relict forms of the Paleozoic that are still alive today are frilled sharks; angel sharks and cow sharks are known from the Jurassic; saw sharks, sawfishes and bullhead sharks date back to the Cretaceous. A special group of evolutionary progressive elasmobranchs are lamnoid sharks, carcharhinids and some lineages of rays. They occupy the highest positions in the trophic pyramids playing a significant role in marine ecosystems. Modern cartilaginous fishes is a prosperous group of fishes inhabiting vast regions of the World Ocean and many freshwater water bodies and comprising about 50 families and several hundred species of sharks, skates, rays and chimaeras. The distinguishing features of the brain of modern sharks, rays and skates are a marked differentiation of all brain regions and a significant development of the telencephalon (Fig. 4). Acute sense of smell, vision, and a sophisticated motor system correlate with the level of development of the corresponding brain regions (olfactory lobes, telencephalon, midbrain and cerebellum). In some sharks, the telencephalization index (the ratio between the weights of the telencephalon and the whole brain) can reach the values comparable to those of higher vertebrates. |
|
Bony fishes (Osteichthyes) |
The bony fishes have originated in the Devonian independently of the cartilaginous fishes. Their ancestors have split early on into the subclasses of Actinopterygii (ray-finned fishes), Polypterigii and Sarcopterygii (fleshy-finned fishes). Ancient ray-finned fishes of the suborder Palaeonisci have already widely radiated in the Paleozoic. For instance, several evolutionary lineages that have originated from one of the lines of Palaeonisci gave rise to the superorder Holostei, whose representatives inhabited freshwater and brackish water bodies of the Mesozoic. This group is now represented by only two families of evolutionary conserved relicts of the Cretaceous: gars and bowfins. The unique feature of the ray-finned fishes is that they have evolved a special everted type of the telencephalon, while retaining the brain structure common to all vertebrates. This type of telencephalic hemispheres lacks lateral ventricles, which are characteristically present in the inverted type of the telencephalon of all remaining vertebrates: from cyclostomes to mammals (Fig. 5).
Fig. 5. Two types of development of the vertebrate telencephalon (after Andreeva, Obukhov, 1999): a – early stages of hemisphere development; b – inverted and c – everted types of the telencephalon; 1 – striatum, 2 – septum; 3-5 – lateral, dorsal and medial zones of the pallium (cortex of the hemispheres), respectively.
At least three lineages of ancient holosteans have evolved in parallel to give rise to the modern bony fishes (superorder Teleostei) (Fig. 6). The teleosts first emerged in small lagoons of the Paleozoic and have begun to gradually replace the holosteans. By the end of the Early Cretaceous they were already widely distributed in seas and freshwaters. The chondrosteans (superorder Chondrostei) have originated from one of the lines of Palaeonisci and evolved by fetalization, i.e. the retention of some traits of early ontogenetic stages to later stages. By the middle Cretaceous, this group of fishes have already split into two branches: sturgeons and paddlefishes (Fig. 7). According to A.I. Karamyan, a significant development of the midbrain and the cerebellum in fishes is associated with the second critical period in the evolution of the vertebrate CNS. In fishes, the cerebellum contains projections from all sensory systems (somatosensory, lateral-line organs, vestibular, optic and auditory), and the midbrain together with the cerebellum become the leading integration centers in the CNS. This type of integration is called the mesenencephalo-cerebellar integration (Fig. 3c). In most fishes, the structures of the forebrain exhibit only a low degree of morpho-functional development and function primarily as an olfactory center. Nevertheless, more recent evidence indicates that the cartilaginous fishes do not conform very well to this evolutionary stage of the nervous system. In a number of sharks, the highest integration center is the telencephalon, just like in higher classes of vertebrates. |
|
The origin of terrestrial vertebrates |
The ancestral group of the bony fishes that gave rise to the land vertebrates (Tetrapoda) were the ancient crossopterygians (fleshy-finned fishes) that belonged to the group known as rhipidistians. As paleontologists currently believe, the climatic conditions of the Devonian (the period when the vertebrates moved to land) were very unstable. This prompted a significant adaptive radiation of the crossopterygians. Some rhipidistians have actively spread into freshwaters, others have begun to live in the marine lagoons, which were at risk of periodic desalination and dry-outs. This may have forced them to adapt to the semi-aquatic lifestyle and prompted the evolution of new abilities, which formed the foundation for their movement to land. It should be noted that many traits that characterize amphibians have been formed in their ancestors still in aquatic or semi-aquatic environments. They proved to be fast in occupying accidentally vacated habitats in water ecosystems replacing the crossopterygians in their original aquatic and to some extent in semi-aquatic environments. Consequently, the adaptation to the habitats that were very unusual for fishes have resulted in gaining novel traits that gave them the abilities to conquer new terrestrial environments. These traits have evolved in parallel in different groups of rhipidistians, and a number of “semi-amphibians” have remained evolutionary dead ends. There was no straight and single evolutionary lineage leading from fishes to the amphibians. |
|
Amphibia |
Modern-day amphibians, comprising the superorder Lissamphibia, are represented by three orders: Urodela, Anura and Apoda. These three groups are thought to have originated from a single ancestral stock, although anurans have descended from a lineage that had been evolving for over 350 million years independently of Urodela and Apoda. They have originated in the Paleozoic from morphologically widely different ancestral groups that lived on different land-masses now located in the Northern and Southern hemispheres, respectively. The transition to the terrestrial lifestyle has stimulated a significant differentiation and growing sophistication of both sense organs and the associated CNS regions. Starting with amphibians, the vertebrate CNS undergoes fundamental evolutionary changes in the degree of development and the hierarchy of different brain regions (Fig. 8). The amphibians together with reptilians represent the third critical stage in the evolution of the vertebrate CNS. When the amphibians moved to land, they have undergone significant transformations in the morpho-functional organization of the body and the CNS compared to the bony fishes. These transformations involved the reduction of some structures associated with the aquatic lifestyle (for instance, lateral-line organs); emergence of new, phylogenetically young communication systems (auditory organs and centers); a more pronounced differentiation of the thalamic regions; formation of the neocortex in the hemispheres; and the establishment of the direct thalamo-cortical connections. All the system of major sensory centers begins to shift from the brainstem, cerebellum and midbrain to the upper regions: diencephalon and telencephalon to form the diencephalon-telencephalic level of integration (Fig. 3d). At this stage, the main direction of cephalization is the progressive evolution of the telencephalon (telencephalization), which reaches its highest level in mammals and humans. |
|
Higher vertebrates (Amniota) |
Amniotes is an evolutionary grade of vertebrates that comprises two phylogenetic lineages, one of which, sauropsids, includes the classes of reptiles (Reptilia) and birds (Aves), and the other, therapsids, consists of the classes Theromorpha and Mammalia (mammals). Evidence from paleontology, comparative morphology and physiology suggests that both lineages could have reached the amniotic level independently, and a number of important amniotic traits might have been formed earlier, at the “amphibian” stage of evolution. The amplification and intensification of function of sense organs and organs of locomotion, behavioral changes in a new and more diversified environment (amniotes have completely occupied terrestrial, aquatic and aerial habitats) were undoubtedly associated with the evolution of a more sophisticated CNS. |
|
Reptiles (Reptilia) |
Among modern-day reptiles, more or less direct descendants of the first reptiles (sublcass Cotylosauria) are turtles and tortoises (subclass Testudines), which stand clearly apart from the remaining reptiles (Fig. 9). Small-sized and highly-mobile insectivore cotylosaurians (which were predatory in their lifestyle) gave rise to another group of reptiles, lepidosaurians (subclass Lepidosauria). Tuatara, which is a relict form of the order Rhynchocephalia that survived from the Mesozoic into the present, lives in relatively simple biotic environments. A wide adaptive radiation of modern lizards (order Lacertilia) have begun in the middle Mesozoic. The modern groups of geckos, agamids, skinks, lacertids, monitor lizards and others represent different and sometimes parallel evolutionary lineages of reptiles. Snakes (order Serpentes) are the phylogenetically youngest order of reptiles that have passed in the Cretaceous through the transitional phase of burrowing ancestors with limbs. One line of evidence for the secondary loss of limbs in snakes is the fact that they retain the vestiges of the entire muscle system of limbs and the corresponding neuronal associations in the spinal cord. In a group of limbless lizards (order Amphisbaenia), the loss of limbs and the transition to the burrowing lifestyle in a simpler environment was also secondary; their ancestors were active and mobile lizards. Archosaurs (subclass Archosauria), or higher reptiles, have also originated from the cotylosaurian ancestors at their “proreptilian” stage of evolution. During the Mesozoic, the members of this large group (the typical representatives of which were dinosaurs) exhibited a complex behavior, and some of them had acquired a certain degree of homoiothermy (the order of flying reptiles, or Pterosauria). The only surviving members of Archosauria, the crocodiles (order Crocodilia), are only moderately high in their organization among the archosaurs. In the modern crocodiles, the most primitive traits are retained by alligators and caimans. The reptiles are of great interest for evolutionary neuromorphology, because the structures of the CNS that would later give rise to the higher brain centers of birds and mammals have first evolved within this class of vertebrates. The hypothesis of the origin of birds (class Aves) from early archosaurs (order Thecodontia) has now been replaced by the hypothesis that the birds have evolved from a group of small-sized predatory dinosaurs of the order Saurischia. These dinosaurs were bipedal (i.e. they walked on two feet) and had a relatively large brain and several other traits common with birds. During the course of vertebrate evolution there were several attempts to conquer the air, some of them proved to be a dead end (for instance, Archeopteryx). One of the intermediate forms between the reptiles and birds called Protoavis was more than 75 million years older than Archeopteryx, but had more bird-like traits than the latter. The evolutionary divergence into carinate and cursorial birds occurred very early, in the Cretaceous, and a rapid radiation of lineages of superordinal and ordinal ranks has begun at about the same period. A special interest to the CNS of birds was incited by the works of L.V. Krushinsky of the Moscow State University who studied the rational behavior in birds and other vertebrates. These studies have shown that the brain of birds, especially that of the family Corvidae, is characterized by a very high level of functional and structural organization and that many birds are equal to mammals in terms of their rational behavior. The birds have highly developed cerebral hemispheres and optic lobes of the midbrain (Fig. 10). |
|
Mammals (Mammalia) |
Two major evolutionary branches of this class have already been formed at the “reptilian” stage and are represented today by prototherians (subclass Prototheria) and therians (subclass Theria). The latter groups comprises marsupials (infraclass Metatheria) and placentals (infraclass Eutheria). Modern prototherians (Platypus and Echidnas) have split from one another very early in their evolution, well back in the Mesozoic Era. They have significantly diverged in their lifestyle from their ancestors and, in addition to retaining some primitive characters, have gained a number of specific adaptions. The marsupials and placentals are two lineages of mammals that have arisen from a single ancestral group of tribotherians (Tribotheria) at the boundary between the early and late Cretaceous. In the marsupials, the evolutionarily conserved relicts of that ancient time are the opossums. The most primitive placental mammals belonged to the now extinct order Protheutheria, which lived from the late Cretaceous to the Paleogene. The earliest forms of Protheutheria gave rise to future edentates and (at least by three parallel lineages) to Afrosoricida, Erinaceomorpha and Soricomorpha, which comprise the order Insectivora. At this stage in the evolution of the mammalian CNS, the area of the neocortex in the cerebral hemispheres has become considerably larger and the association areas have been formed. The thalamus and the telencephalic nuclei have reached a significant differentiation. Since a crucial role in the complex patterns of behaviour in insectivores and rodents is played by the striatum, this stage is known as the striato-cortical integration and represents the fourth critical stage in the CNS evolution (Fig. 3e). At the end of the Cretaceous, the evolutionary offshoots of protheutherians gave rise to first primates, chiropterans and flying lemurs (Dermoptera). Groups of the protheutherians that were the ancestors of rodents were probably already phylogenetically remote from those forms, which were ancestral to the modern lagomorphs. One of the major groups of placentals that have originated from protheutherians, were ancient ungulates (order Condylarthra), which date back to the middle of the late Cretaceous. By shifting to eating plants, they significantly increased their numbers, spread across the lands and gave a wide adaptive radiation. One group of ancient ungulates gave rise to the orders Tubulidentata, Hyracoidea, Sirenia, Proboscidea, Artiodactyla, Tylopoda, Perissodactyla, Cetacea, etc. Even within the same order, different groups could have evolved from different ancestral forms (for instance, toothed and baleen whales). The ancestors of Carnivora, the largest order of Placentalia, were protheutherians or very early ancient ungulates, which shifted to the predatory lifestyle. The canines and viverrids are close in their general organization to the their Paleogene ancestors, while the felines are the most derived and specialized forms of carnivores. Ancient carnivores that moved back to water may have been the ancestors of the pinnipeds (seals and their allies), in which the eared seals are probably most similar to the early forms of pinnipeds that lived at the boundary between the Paleogene and Neogene. In the higher orders of mammals (carnivores, primates, proboscideans, etc.), the tendency towards brain telencephalization has reached its highest level, which was reflected in the significant increase of the overall size of the cerebral hemispheres and the area of the neocortex, and in the increase of the complexity of its internal structure. This led to the evolution of the complex forms of neural activity including the rational reasoning and thinking (in humans). This stage was viewed by A.I. Karamyan as the fifth and the highest critical stage in brain evolution. Higher integration centers become concentrated in the phylogenetically young and progressively evolving zones of the neocortex: frontal and parietal cortices. This evolutionary stage is called the neocortical integration (Fig. 3f). |
|
Conclusion |
The division of the brain into five regions that have originated from the brain vesicles is a defining feature of all groups of vertebrates from cyclostomes to mammals and humans; however, the structure and functions of these regions have undergone significant transformations in evolution. The present evidence challenges the traditional scenarios portraying the evolution as a linear process “from lower to higher groups”. In each group of modern vertebrates, there are different types of organization of the nerve centers and the brain as a whole, which have been evolving in parallel and independently from one another. In this respect, even the human brain is simply one of many possible and actually realized types of the CNS architecture. In many cases, the evolution of the major vertebrate groups not only proceeded along independent and parallel lines, but also progressed at different rates and intervals. This “mosaic” pattern of animal evolution was undoubtedly reflected in the structure of their nervous system. Important goals of evolutionary neuromorphology are the study of the pathways along which the nervous system and the brain were evolving, the factors influencing their evolution, and the identification of analogies/homologies in the regions of the nervous system in different animal species. All of this provides the foundation for the correct interpretation of the data obtained in various neurobiological studies of vertebrate species, many of which are used as models for studying the whole range of problems posed by physiology, medicine, toxicology, developmental biology, etc. |
|
Recommended literature |
|